Solar-Cosmic Activity
The ionosphere is the inner part of the upper atmosphere, extending from about 50 km up to the upper ion transition level (varying between 1000 and 2000 km altitude), which is being ionised by the solar radiation. Most of the ionisation is produced by solar X-ray and ultraviolet radiation and by corpuscular radiation from the Sun.
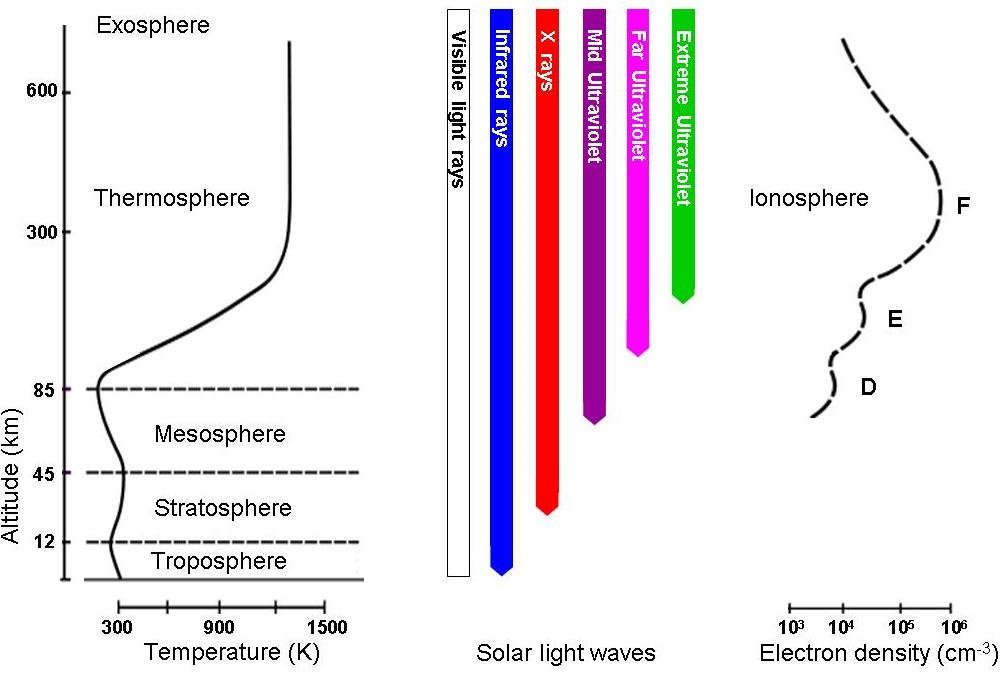
Monitoring the solar activity is of paramount importance for understanding and modelling the ionospheric behaviour/effects since the ionosphere is produced mainly via the ionisation effect of the solar electromagnetic radiation (Akasofu and Chapman, 1972; Hargreaves, J.K., 1992). Since the intensity of the solar emissions varies with time, quantification of the solar activity is therefore required for research purposes and development of ionospheric models. Solar activity indices are produced (and also needed) by various ionosphere/space weather monitoring services. Currently we use the solar 10.7 cm radio flux index (F10.7) as provided by the NOAA Space Weather Prediction Center.
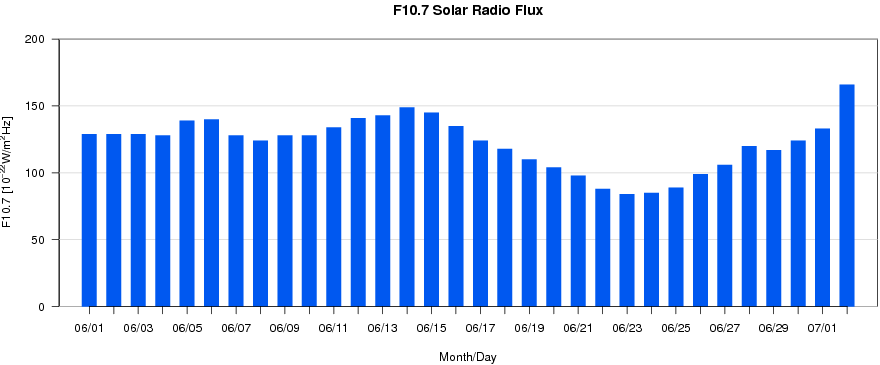
Here is a link to the current F10.7 index values.
The interplanetary space is continuously traversed by energetic nuclear particles called cosmic rays (e.g., Stankov et al., 2012). The origin of the cosmic rays is still not definitely determined—some certainly originate from the Sun and other stars but others are believed to be associated with various energetic processes in the galaxy. Cosmic rays consist mostly of protons (~94%) and helium nuclei (~5.5%), with few nuclei of heavier elements (galactic component of the cosmic rays). By colliding with the components of the Earth's atmosphere, new atomic particles of high energies are created (i.e. the so-called “high-altitude radiation”).
The solar activity alters the intensity and energy spectrum of the galactic cosmic rays that enter the solar system—when the Sun is active, fewer galactic cosmic rays reach the Earth than during times when the Sun is quiet. As a result, the galactic cosmic ray intensity follows an approximately 11-year cycle like the solar activity but in the opposite direction: the higher the solar activity, the lower is the cosmic radiation level, and vice versa.
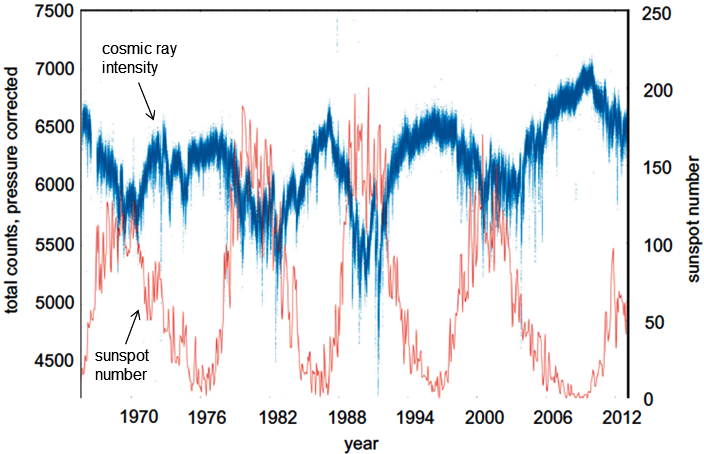
The Earth’s magnetic field (partially) deflects the cosmic rays from the atmosphere—this shielding is strongest at the geomagnetic equator and weakest at the geomagnetic poles.
Occasionally, the Sun emits particles of sufficient energy and intensity to sharply raise the radiation levels on the Earth's surface, an event called “Ground Level Enhancement” (GLE). Also, during massive solar coronal mass ejections (CMEs), the CME’s enormous quantity of mass and energy is able to substantially suppress the intensity of galactic cosmic rays, an event called “Forbush Decrease” (FD).
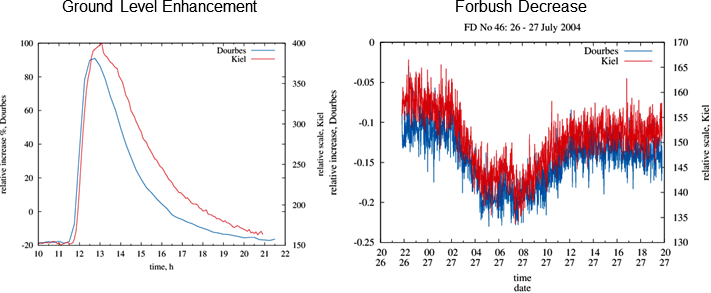
The standard instrument to measure the cosmic ray activity from the ground is the neutron monitor. At the RMI Geophysical Centre in Dourbes, a 9-tube NM64 neutron monitor has been in continuous operation since 1965 (Jodogne, 1970; Bossy and Jodogne, 1972). The neutron monitor provides measurements of the secondary neutron component of the cosmic rays on the ground. After pressure correction, this component would follow closely the primary cosmic rays intensity, i.e. would reproduce the variations of the cosmic rays intensity as is high above the station. However, before any useful information can be extracted from the raw neutron monitor measurements, missing records need to be recovered and the data has to be filtered and corrected. For space weather applications, all these steps has to be carried out in real time and answer to a strong requirements among which — to minimize the introduction of spurious data (spikes) and to eliminate any possibility for removal of correct original data. For the purpose, a Real Time Automatic Data Correction (RTADC) algorithm has been developed (Sapundjiev et al., 2013, 2014).
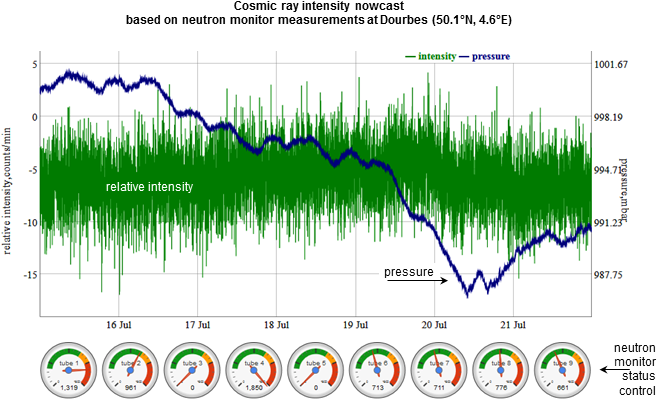
Here is a link to the implemented RTADC monitoring service based on measurements from the neutron monitor in Dourbes.
Real-time data from networked neutron monitors around the globe can be successfully used for prompt identification of major solar events, including the abovementioned GLEs and FDs, that may cause adverse effects on sophisticated modern-day technological systems.
References:
Akasofu, S.I., Chapman, S. (1972): Solar-Terrestrial Physics. Oxford University Press, Oxford, UK.
Hargreaves, J.K. (1992): The solar-terrestrial environment. Cambridge University Press, Cambridge, UK.
Jodogne, J.C. (1970): Mesure permanente de la multiplicité du rayonnement cosmique secondaire dans un moniteur NM64. Annales de la Soc. Scient. de Bruxelles, Vol.84, No.11, pp.253–256.
Bossy, L.G., J.C. Jodogne (1972): Utilisation à l'I.R.M. d'un mini-ordinateur dans les systèmes de mesures ionosphériques et du rayonnement cosmique. Bull. Sci. AIM, Vol.85, No.4, pp.245–254.
Stankov, S., J.C. Jodogne, S. Spassov (2012): Cosmic Rays — A Review. Pres. RMI Conference on Cosmic Rays, 18 Apr 2012, Brussels, Belgium. (download)
Sapundjiev, D., S. Stankov, J.C. Jodogne (2013): Data analysis of Dourbes neutron monitor data for solar events forecast. Proc. European Space Weather Week (ESWW), 18–22 Nov 2013, Antwerp, Belgium. (download)
Sapundjiev, D., M. Nemry, S. M. Stankov, J.C. Jodogne (2014): Data reduction and correction algorithm for digital real-time processing of cosmic ray measurements: NM64 monitoring at Dourbes. Advances in Space Research, Vol.53, No.1, pp.71-76. (doi:10.1016/j.asr.2013.09.037 , download)